Choosing the optimal region of the electromagnetic spectrum for your application is a challenging decision but essential for optimum system performance. Infrared radiation or thermal imaging is used in night vision devices to detect heat emitted by objects in the scene. Thermal infrared cameras are available in several wavebands, cooled medium wave infrared, and Long wave infrared. Long wave infrared has two different solutions, one is cooled, while the other is uncooled. Uncooled LWIR cameras are lower cost, but not as sensitive as their cooled counterparts. When considering MWIR and LWIR waveband, it is important to note that medium wave cameras are generally much smaller than long wave cameras and generally offer a higher level of performance.
When considering size, cost and performance, mid wave infrared cameras are best for long-range applications for ground, airborne and maritime applications. Uncooled long-wave infrared cameras do not require cryogenic coolers, so can be made smaller, but are limited to shorter, medium ranges making them better suited to tactical applications. Uncooled Long-wave infrared technology provides significant cost advantages for shorter-range applications, such hand held night vision, thermal sights and vehicle sights or situational awareness applications such as Drivers vision enhancers (DVE).
Understanding infrared camera technology can be challenging. Sensor and camera manufacturers often make impressive claims for sensitivity, resolution and operational life, but there are also many different assumptions that accompany these metrics making it difficult for platform integrators to compare products equally. We strongly recommend real-world side-by-side comparison of infrared cameras for your chosen application to determine the best choice.
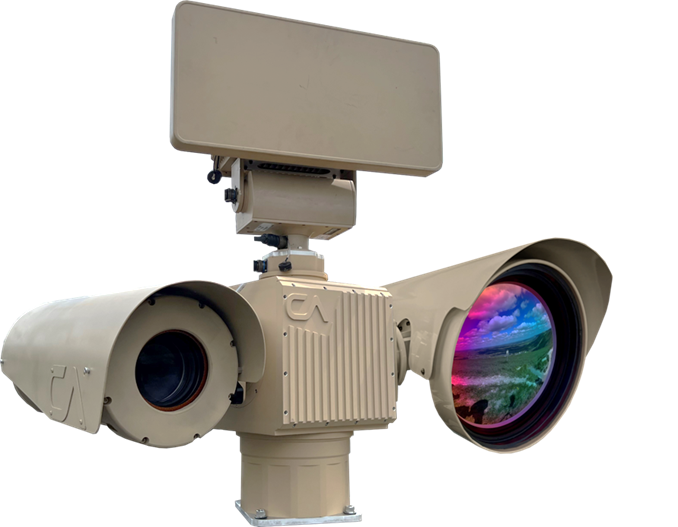
Differences between a Visible Light and an Infrared Imaging?
Day cameras capture visible light reflected within electromagnetic spectrum that is visible to the human eye (Visible light). Infrared cameras, enable you to detect heat radiation (infrared light) based on the temperature of objects in the scene, both during the day and night giving 24/7 imaging in total darkness.
Short-wave infrared (SWIR) differs from the thermal infrared wavebands as it measures reflected radiation in a similar way to visible light sensors. Short wave infrared offers advantages over visible light as the longer wavelength enables improved penetration of fog and haze. Sensors operating in the Long-wave infrared (LWIR) and mid-wave infrared (MWIR) capture electromagnetic infrared radiation or thermal energy (heat). This key difference enables infrared cameras to perform in low ambient light conditions or indeed total darkness where there is insufficient visible light available in the visible spectrum.
For the system integrator, sensor waveband options include visible light, short-wave infrared, mid-wave infrared, and long-wave infrared. This guide focuses on the differences between MWIR and LWIR thermal imaging to help you decide which waveband best suits your needs for the infrared camera.
1. Seeing at Night: Detecting Infrared Light (Radiation)
The infrared sensor technology chosen greatly influences the performance, cost, and size of the camera or system. Infrared wavelengths allow detection of thermal emissions from celestial objects, people, and machinery. In contrast, visible light sensors rely solely on ambient illumination to form their imagery. It is crucial to understand the advantages and disadvantages of different regions of the infrared spectrum to make an informed decision about the best sensor for your application.
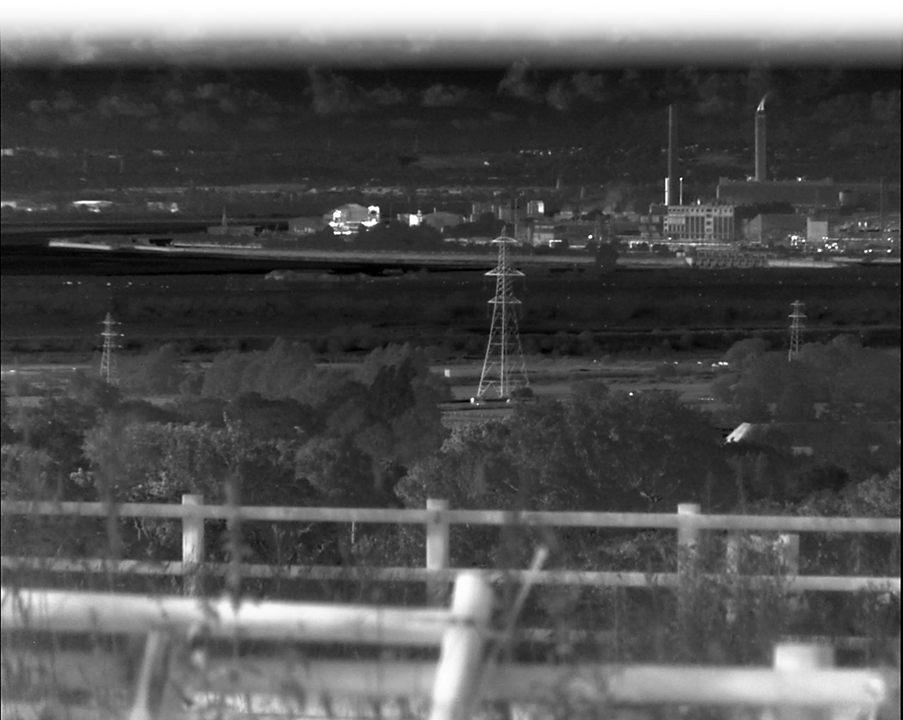
What are Infrared Waves Used For?
MWIR Infrared (MWIR)
Mid-wave infrared (MWIR) cameras operate in the 3-5µm region of the electromagnetic spectrum and is ideal for detecting thermal radiation emitted by objects, even in low-light conditions or total darkness. MWIR cameras are commonly used for border/perimeter security, maritime vessel monitoring, and protecting critical infrastructure. MWIR detectors are also valuable for tracking celestial objects and mapping thermal emissions from objects like cool stars in the solar system. MWIR is ideal for airports, security, oil and gas and critical infrastructure protection. Mid-infrared cameras are typically less susceptible to atmospheric conditions (precipitation and humidity) than long-wavelength cameras.
LWIR Infrared (LWIR)
Long-wave infrared (LWIR) cameras operates in the 8-14µm region is perfect for industrial inspections, surveillance, and night vision. Long-wavelength infrared detectors can accurately detect small temperature differences or thermal signatures, making them ideal for applications such as night vision, thermal weapon sights, surveillance, industrial inspection, and heat loss detection.
What is the difference between cooled and uncooled?
Infrared cameras convert infrared light to an infrared image so that you can see infrared light visually. There are two main sensor types used for thermal infrared detection: cooled and uncooled.
Cooled sensors are photon detectors that operate at cryogenic temperatures to improve the material's sensitivity to infrared light by reducing thermal noise. They are often used in military applications as they have exceptional sensitivity, making them suitable for long-range detection of infrared radiation. Mid wave infrared (MWIR) and longwave infrared (LWIR) detectors operate with greater sensitivity when they are cooled.
In contrast, uncooled sensors, often known as microbolometers, measure temperature changes in the infrared waveband without the need for cryogenic cooling. They are a cost effective alternative to using cooled detectors. Uncooled detectors do not have the sensitivity of cooled detectors, but they have reduced size weight and power requirements so are well suited for man portable applications.
How do Microbolometer Infrared Detectors Work?
Microbolometers are uncooled detectors that change resistance based on temperature differences, detecting variations as small as 0.02°C or 20mK. While microbolometers are widely used in night vision goggles and handheld thermal cameras, their sensitivity can vary depending on the sensor material, pixel size and pixel geometry. Infrared images can vary dramatically from sensor to sensor, even though at first glance they may appear equivalent.
How Do Cryogenically Cooled Sensors Work?
Both long-wavelength infrared and mid-wave infrared sensors can have their sensitivity to infrared light enhanced by adding a cooling mechanism. Cooled infrared detectors are typically made from Mercury Cadmium Telluride (MCT) or Indium Antimonide (InSb), which are both sensitive to small temperature differences. The doping of the material determines the precise waveband of sensitivity. MCT can be doped to operate in the MWIR or the LWIR. Newer Type-II strained superlattice (T2SL) detectors have an increased ability to tune the waveband in the SWIR, MWIR and LWIR wavebands, T2SL, MCT and InSb cooled sensors are commonly used in military applications. Cooled sensors detect temperature by measuring the excitation of electrons within the sensor material, with cooling reducing noise in the infrared signal creating a clearer image. T2SL sensors and some MCT devices can operate at higher temperatures (approximately 150 K), this class of detector is called HOT as they operate at a higher temperature than a classical cooled devices (77K). HOT detectors operate at a temperature between a traditional cooled and an uncooled sensor.
What is Noise Equivalent Temperature Difference (NETD)?
NETD is a critical factor in determining how well a thermal imager can detect small temperature differences. For example, when viewing a person with a temperature of ~310K against a background at ~290K, the thermal imager detects the 20K difference. However, as temperatures become more similar, the task becomes more difficult, making NETD a key performance measure. NETD values for infrared detectors vary based on sensor material and pixel size, real-world testing is essential to ensure reliable performance since specification alone may not fully represent the sensor.
There are different definitions of NETD relating to temporal and spatial noise mechanisms in the sensor, so it is important to understand what is being quoted. For bolometers, NETD values vary with yield, pixel pitch, and frame rate so typical values are closer to 40-50 mK, although some some sensors offer 20 mK sensitivity with frame averaging (which is a trade-off with frame rate). For cooled detectors, NETD values vary between 15 and 35 mk depending on the chemistry that is used and the operational temperature.
We recommend comparison in real world due to Environmental factors
It is convention to specify NETD at F/1, 30 Hz for uncooled devices which is not necessarily how the sensor will be used. There are several sensor materials used for microbolometers, the most widely used is Vanadium Oxide (VOx) but Titanium Oxide (TiOx) and Amorphous Silicon (a: Si) are also offered by some manufacturers. The sensitivity of the microbolometer depends on the sensor material, but also on the size and the geometry of the pixel. There can be meaningful differences between sensor NETD from different manufacturers. Infrared images can vary dramatically from sensor to sensor even though at first glance they may appear to be equivalent. This is why we recommend comparison in real world environments.
How Do Cooled Sensors for the Mid Infrared Region and Long Infrared Region Work?
Both long wavelength infrared and mid wave infrared sensor material can have sensitivity to infrared light enhanced by adding a cooling mechanism. Cooled sensors measure temperature by excitation of electrons in the sensor material, 'jumping' from the valence band to a conduction band. When thermal radiation is present, electrons can ‘jump’ over the energy gap between the two bands and a current flows. Cooling is required to avoid thermally excited electrons being detected and reduces noise in the image. Commonly used infrared spectrum detection materials are:
-
Mercury Cadmium Telluride (MCT)
-
Indium Antimonide (InSb)
-
Indium Arsenide (InAs)/ Gallium Antimony (GaSb)
-
Type II superlattice (T2SL)
-
Quantum Well Infrared Photodetectors (QWIP’s).
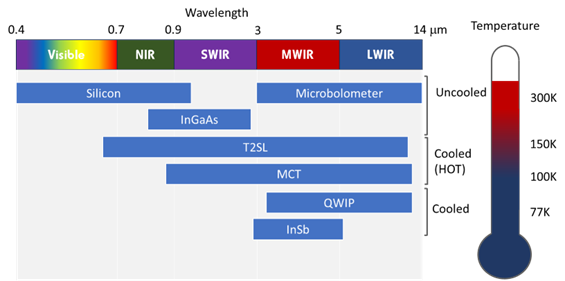
Figure 1 infrared Wavelength and Temperature of Operation for Commonly Used Sensor Materials
For MWIR light detection, most currently fielded systems today use either MCT or InSb detectors. InSb requires cryogenic cooling down to 77 K for optimum operation. MCT can operate between 77 and 150 K depending on the doping of the material, but there is a trade-off with sensitivity (NETD). T2SL sensors are a newer technology compared to InSb and MCT. Fundamentally, T2SL is capable of comparable performance with the traditional cooled materials but also offers the ability to operate at higher temperatures (up to 150K) and can be tuned for specific wavebands or even multiple waveband operation. MCT is also highly tunable and dual-band (MWIR/LWIR) and (SWIR/MWIR) sensors are available from some manufacturers. QWIP sensors operate most effectively in the LWIR. The ‘party trick’ for QWIP sensors is that they are sensitive to the polarization of incoming radiation so can offer enhanced levels of discrimination.
Currently Available Infrared Sensors
There are a growing number of thermal infrared sensors from manufacturers all over the world. A representative list of such devices is given in Figure 2 including both cooled and uncooled sensors.
Thermal infrared sensors are available in high-definition formats varying from 1280x720 up to 2560 x 2048 pixels. Most high-end camera systems (including some hand-held systems) use the SXGA 1280 x 1024 format. Modern thermal infrared detectors are available with pixel pitches ranging from 17 mm to 5 mm. Larger pixels collect more infrared energy so fundamentally have higher sensitivity and lower NETD. Significant technological developments are required to enhance sensitivity for smaller pixels.
Detectors with the ultra-small 5 mm pixels are comparable in size to the wavelength they are collecting so require higher aperture optics to maintain sensitivity and sharp imagery.
Smaller pixels enable cameras with shorter focal lengths to be realized, but often this focal length reduction is offset by increased aperture. We will explore these trades in more detail.
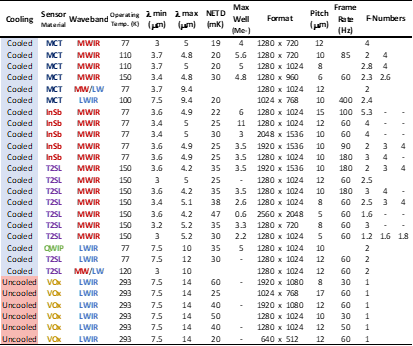
Figure 2 Currently Available Infrared Sensors
Infrared Camera Performance Depends on the Electromagnetic Spectrum Detected
The performance of an infrared camera is determined by the sensor and the resolution in object space. For a given sensor format, the corresponding field of view can be easily calculated. The aperture varies as a function of resolution and field of view as show in data below that compares long-wavelength infrared (LWIR) and medium-wavelength infrared (MWIR) cameras.
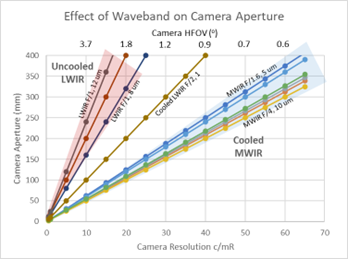
Figure 3 Effect on Waveband and Sensor Choice on Camera Aperture
Because sensors have defined f-numbers (in the case of cooled devices) or in the case of uncooled, the need for low NETD dictates an f-number of approximately 1.0, we can illustrate how camera aperture varies as a function of camera resolution/field of view (Figure 4).
We can see that uncooled LWIR cameras have large apertures for modest camera resolutions. For an SXGA sensor, a 3.7o HFOV will have an aperture of 160 to 240 mm depending on the pixel pitch.
By comparison, a MWIR-cooled camera will have an aperture of 50 to 63 mm for the same field of view and resolution. As most LWIR sensors are uncooled (see Figure 2), the practical limit for LWIR cameras is in the range of 200 to 300 mm focal length. Cooled LWIR sensors do exist and are usually reserved for military vehicle-based applications. These fall between uncooled LWIR and Cooled MWIR in terms of the camera lens aperture. For our example of 3.7o HFOV, a LWIR cooled system would have an aperture of 100 mm. The relative apertures for equivalent-resolution cameras are illustrated in Figure 4.
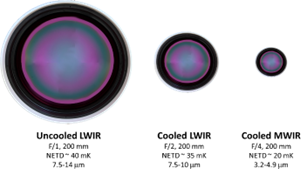
Figure 4 Camera Apertures for Equivalent Resolution
Even though the resolution of the cameras is the same, the performance of the cooled systems will be superior for long-range applications due to increased sensitivity and reduced signal-to-noise in real-world conditions. In our example above the cooled MWIR NETD is a 50% improvement over the uncooled LWIR system. The NETD of the uncooled sensor requires a wide range of wavelengths (7.5-14 mm) to collect energy from the scene.
In real-world conditions, these wavelengths are attenuated at longer ranges which impacts system performance. The cooled sensors use narrower wavebands so are less affected by atmospheric absorption (depending on the specific climatic conditions).
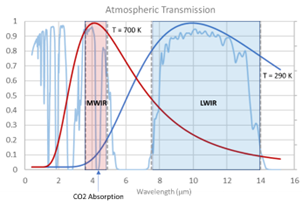
Figure 5 Atmospheric Transmission (US 1976)
Atmospheric Considerations when Detecting Emitted Infrared Radiation
Atmospheric conditions play a significant role in the performance of infrared imagers. Infrared waves are attenuated by water vapor in the air. As a general rule, LWIR is better suited to colder climates, while MWIR has benefits in warmer climates. In maritime environments, mid-infrared cameras often outperform broader waveband systems in polluted atmospheres.
Atmospheric conditions play a significant role in the performance of infrared or thermal imaging sensors. Infrared or thermal imagers are effective at penetrating smoke and battlefield obscurants but they are attenuated by water vapor in the air. As a general rule, LWIR is better suited to colder climates whereas MWIR has benefits in warmer climates. The range of LWIR thermal imagers is often significantly limited by the presence of water vapor, MWIR thermal imagers are less affected and therefore ideal for maritime infrared applications. The specific wavelength or waveband of the camera and the NETD is particularly important when evaluating real-world performance. For example, in some maritime environments and hot polluted atmospheres, a narrower waveband MWIR camera can have some advantages over a broader waveband system. The preferred wavebands for use in different parts of the world are summarized below.
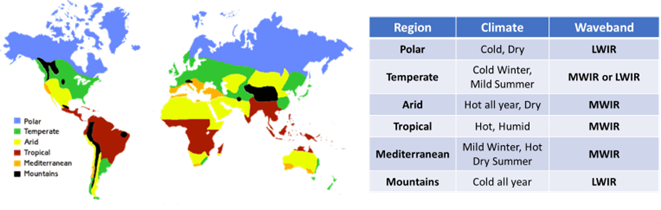
Figure 6 World Climate Map (Courtesy UK Met Office) and preferred waveband by geographic region.
Zoom, Focus and Athermalization
Infrared surveillance cameras typically have a zoom capability of between 5x-30x continuous zoom. Uncooled systems have more modest zoom ratios as it is more challenging to design optics with a high zoom ratio at the higher aperture required by uncooled sensors. In contrast, cooled sensors operating with lower apertures enable higher zoom ratios to be achieved.
The optical properties of infrared materials vary dramatically with just a few degrees of change in ambient temperature. It is for this reason that thermal cameras have athermalization capability, typically realized by having several temperature sensors and a temperature-dependent zoom/focus automatically compensating for temperature changes.
Focus capability is necessary in addition to zoom so that objects can be focused correctly; auto-focus is a useful feature.
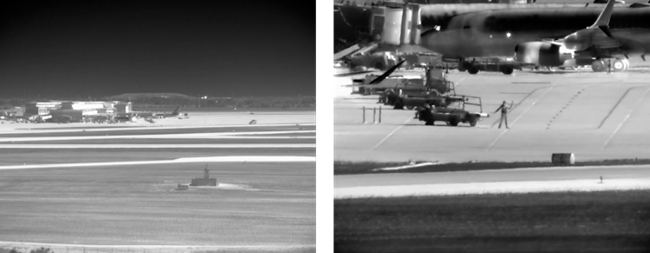
Figure 7 Illustration of the benefit of a zoom lens: Examples uses a 12 x zoom from WFOV to NFOV
Electronics and Video Processing
Camera Calibration
Whichever sensor you choose, the camera must be calibrated for the temperature range that it will be used over. Simply placing a lens in front of a detector will not deliver optimum performance as there will be image non-uniformities and the sensor must be calibrated for the actual lens that is being used. Clear Align has a unique process to calibrate our cameras in a thermal chamber over a range of temperatures to ensure optimum performance in all conditions.
Non-Uniformity Calibration (NUC)
Once your camera is nominally calibrated, it will requires a non-uniformity calibration (NUC). Calibrated cameras still have residual pixel-to-pixel errors which vary depending on numerous external factors. To correct these residual errors a NUC is performed. A uniform surface or shutter is used to normalize the response of the detector pixels producing a well-corrected image. It is common to perform a NUC when the camera is switched on and periodically during operation, as pixel offsets drifts over time.
Video Processing
Uncooled sensor cores often have built-in video processing such that a corrected video signal is produced without the need for further video processing. The functionality of the image processing does vary between providers, so it is important to understand whether the features offered meet your system requirements.
Typical features include:
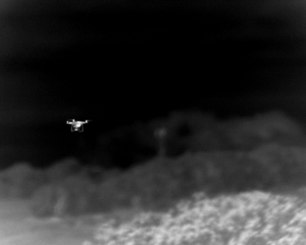
Figure 8 Thermal Image of a DJI Phantom (Drone) at 250 m
Cooled sensors require additional video processing boards as this capability is seldom offered as part of the integrated detector cooler assembly (IDCA). Numerous 3rd party solutions are offered or many camera OEM’s use their proprietary video processing solutions which can add some additional enhancements to the camera performance. Figure 7 illustrates a camera that has sufficient control to optimize the thermal contrast of the drone against the cold background of the sky. Many cameras find imaging of drone targets challenging due to the need to see small temperature differences over the drone against the cold sky in the background.
Other useful advanced video processing capabilities used for cooled cameras include:
-
Turbulence mitigation
-
Electronic stabilization
-
Image sharpening
-
Super-resolution
Frame Rates
Uncooled sensors gain energy from the scene by using a broad waveband and by increasing the stare time of the pixel. The longer the stare-time, the more scene energy that can be collected. The thermal time constant for a typical bolometer is 7-12 ms. For 60 Hz operation, the time constant must be less than 16.67 ms as otherwise there will be image smearing. Some camera cores use successive frames to increase the energy collected by the detector which improves the NETD, but this is at the expense of image smearing.
Cooled detectors have an integration time which is defined by the charge handling capacity of the capacitors used to store the charge of each pixel in the Read Out Integrated Circuit (ROIC). For cooled detectors, integration times are typically of the order of microseconds, so they can operate at much higher frame rates than uncooled sensors. Frame rates of 100-400 Hz are readily achievable which is useful for detecting fast events such as hostile fire.
Video Standards
A fully digital architecture enables high-quality, high-definition video to be delivered to the host platform. Preferred video formats include HD-SDI and h.264/265 over Gigabit Ethernet.
Conclusion
Uncooled LWIR cameras require ‘fast’ ~F/1 optics to deliver acceptable levels of performance. This technology is best suited to applications requiring low Size Weight, Power, and Cost, (SWAP-C) for example small drones, hand-held equipment, and some vehicle applications.
Cooled MWIR cameras are the best-suited thermal imagers for long-range applications such as Border Surveillance, Critical Infrastructure Protection, Maritime, and Counter Unmanned Air Systems (C-UAS). Thermal imagers based on sensors with 8-10 mm pitch are optimum for these applications to maximize range and minimize size.
Cooled MWIR cameras with ultra-small 5-6 um pixels offer the ability to create large array formats (e.g. 2560x2048 and larger). Using these small pitch detectors requires larger optical apertures (lower F/#’s), so these sensors are best suited for short to medium-range applications with shorter focal lengths.
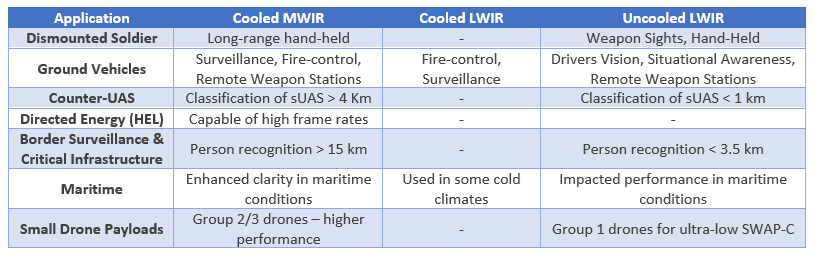
Selecting the best LWIR and MWIR Cameras from Clear Align
A LWIR infrared image excels at detecting and mapping heat, while a MWIR image is preferred for applications requiring higher resolution and clarity, especially in complex environments. As you consider your options for infrared wavelengths beyond the visible light spectrum it is key to have an expert to support your selection process.
Clear Align is happy to support you from the visible spectrum to the infrared in selecting and building the right solution.